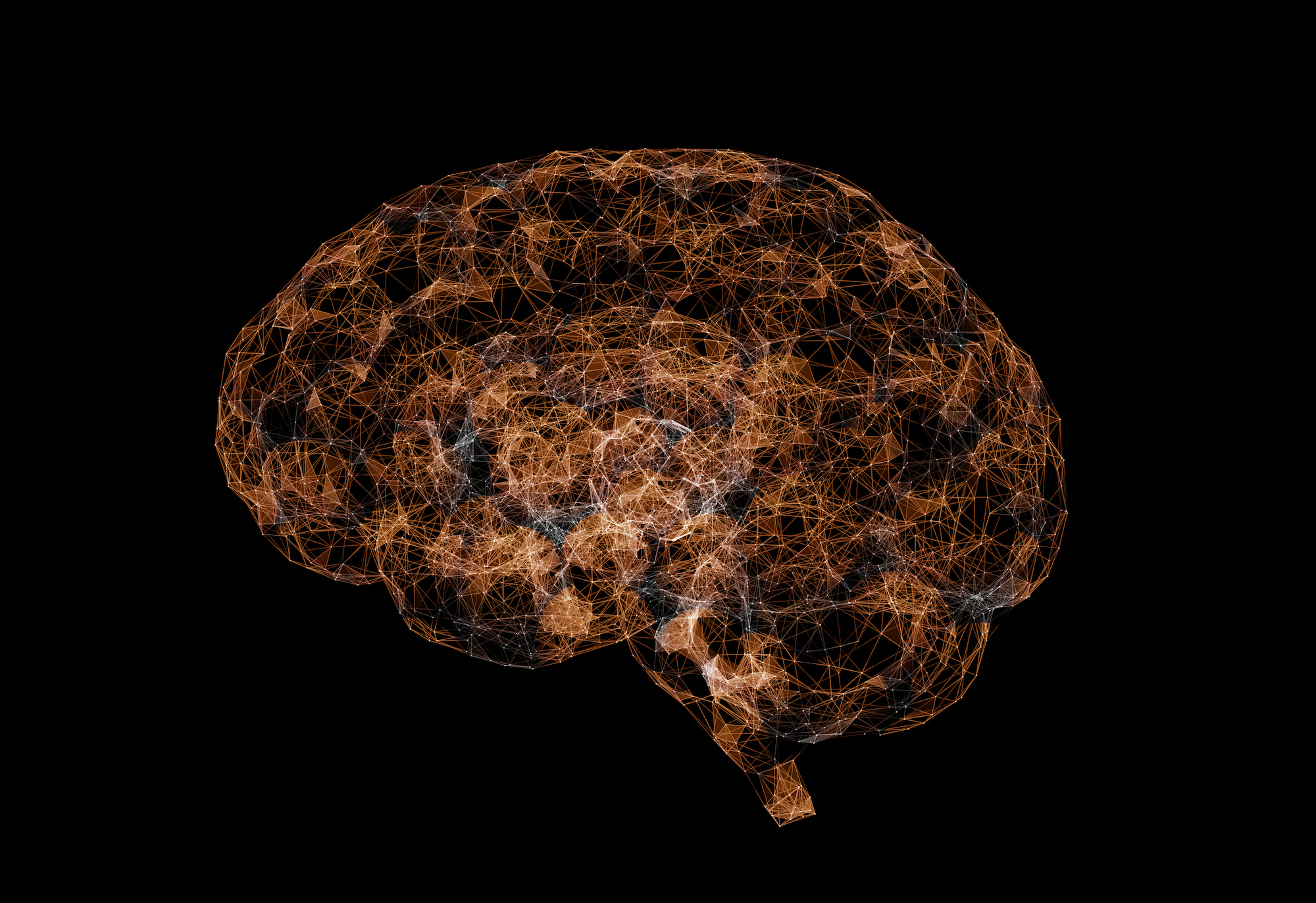
A long-standing mystery in science is how the more than 86 billion individual neurons work together to create a network that forms the basis of who we are, including every human thought, emotion, and behavior.
Mapping these constellations of cells and discovering their function have been long-standing goals of scores of 21st century molecular cartographers working worldwide as part of the National Institutes of Health’s Brain Initiative Cell Census Network project. The overarching purpose of the atlas is to aid in the development of neuroscience research. The hope is that it will allow scientists to gain a better understanding of brain diseases and hard-to-solve medical mysteries behind disorders such as autism and depression.
Now, a series of new studies published in Science Advances has revealed the widespread profiles of the inner molecular workings of the brain at an unprecedented level and scale.
As part of the effort to better understand the evolution of the brains in people and animals, a research team led by scientists at the University of Pennsylvania, Arizona State University, the University of Washington, and the Brotman Baty Institute generated the world’s largest primate brain-wide atlas.
“These data will also provide a critical and much-needed map of complex human-relevant social behavior and disease, as well as the substrate for identifying similarities and differences in these cells and networks across species,” says senior co-author Michael Platt, a Penn Integrates Knowledge Professor.
The team’s goal was to identify and examine many of the brain cells (neurons and non-neurons) and perform a complete molecular analysis using state-of-the-art single-cell technologies.
“Mapping what cells are where and what they do in the adult primate brain is crucial both for understanding the evolution of human cognition and behavior as well as for identifying what happens when things go wrong and lead to neurological disorders,” says senior co-author Noah Snyder-Mackler of Arizona State University (ASU).
Studying data from 30 different brain regions, the researchers meticulously constructed a comprehensive atlas, cell by cell. In total, this atlas represents a 4.2-million-cell map of the adult primate brain. For each cell nucleus, they analyzed gene expression (2.58 million transcriptomes) and DNA gene regulatory regions (1.59 million epigenomes).
The researchers say this “multi-omic” approach, which draws from multiple “ome” datasets, such as transcriptomes and epigenomes, enabled them to delve into the molecular blueprints defining unique brain cell types, offering a deeper understanding and the potential for detailed cellular manipulation.
“Our data, which we have made open and available to the scientific community and broader public, represent the largest and most comprehensive multimodal molecular atlas in a primate to date, and are crucial for exploring how the many cells of the brain come together to give rise to the behavioral complexity of primates including humans,” says senior co-author Jay Shendure of the University of Washington, director of the Brotman Baty Institute.
From the gene expression profiles, the researchers were able to identify hundreds of molecularly distinct brain-cell types. They also found that cell composition differed extensively across the brain, revealing cellular signatures of region-specific functions, from the neurotransmitters involved in brain cell communication to support cells that help feed and protect the brain from diseases like Alzheimer’s.
The researchers used their data to investigate 53 phenotypes relevant to risk of neurological diseases, disorders, syndromes, behaviors, or other traits. Their results captured known roles of cell classes implicated in neurological diseases, including cells linked to cardioembolic stroke or ischemic stroke, the leading cause of neurological death in people.
They also found that genes linked to Alzheimer’s disease tended to fall within DNA regulatory regions that are only accessible in microglia—the brain’s primary immune cell that protects neurons—consistent with the prominent role of microglia proliferation and activation in Alzheimer’s disease found from genome-wide association studies (GWAS).
“We identified numerous associations between genetic risk for neurological disorders and the epigenomic states of specific cell types, some of which had yet to be connected,” says co-lead author Kenneth Chiou of ASU. Many of the new regulatory regions they found allowed the team to explore the genetic architecture of neurological disease risk at the cellular level.
Another type of cell class, basket cells, were enriched for the greatest number of GWAS phenotypes, including disorders such as schizophrenia, bipolar disorder, major depressive disorder and, most strongly, epilepsy. They also found enrichment of Parkinson’s disease-associated sites among open regions in the glial OPC, oligodendrocyte, and astrocyte cell classes.
Finally, they found that heritable sites associated with attention deficit/hyperactivity disorder (ADHD) in their analysis were enriched only among open regions of medium spiny neurons. Medium spiny neurons have been linked to behavioral hyperactivity and disrupted attention via activation of astrocyte-mediated synaptogenesis. Their results suggest that medium spiny neurons may be a promising new target for future ADHD-related study.
Together, the multi-omic atlas now provides an open resource to the worldwide research community for further investigations into the evolution of the human brain and identifying novel targets for disease interventions.
Michael Platt is a Penn Integrates Knowledge Professor and the James S. Riepe University Professor, professor of neuroscience, professor of psychology, and professor of marketin, with appointments in the Perelman School of Medicine, School of Arts & Sciences, and Wharton School at the University of Pennsylvania.
Other authors include Martin Bohlen, Sébastien Tremblay, and Michael Montague of Penn Xingfan Huang, Lea Starita, and Jay Shendure, of the University of Washington; Kenneth Chiou, Trisha Zintel, Madeline Andrews, and Noah Snyder-Mackler of Arizona State University; Jay Shendure of the Allen Discovery Center for Cell Lineage Tracing; Alex DeCasien of the U.S. Department of Health and Human Services (National Institute of Mental Health); Melween Martinez of the University of Puerto Rico; Cailyn Spurrell and Aishwarya Anand Gogate of the Seattle Children's Research Institute; and Lea Starita, Cailyn Spurrell, Aishwarya Anand Gogate, and Diana O'Day of the Brotman Baty Institute for Precision Medicine.
This research was supported by the National Institute of Health (grants U01-MH121260, R01-AG060931, R00-AG051764, R01-HG010632, R01-MH118203, R01-MH096875,R37-MH109728, R21-AG073958, R01-MH108627, R56-AG071023, R56-MH122819, T32-AG000057, K99-AG075241, and P40-OD012217); National Science Foundation (grants TIP-2110037 and BCS-1800558); Kaufman Foundation (grant KA2019-105548); Canada Research Chairs (Grant 950-231257); and Canada Research Coordinating Committee (Grant NFRFE-2018-02159).